Genomic sequencing has the potential to expand newborn screening programs, identifying many more treatable conditions before symptom onset.
What is newborn screening?
Newborn screening is a highly effective and successful public health initiative to identify babies with medical conditions that can be effectively treated before symptoms start.¹ Newborn screening is governed independently by each state and territory of Australia and screens 99% of babies. Screening currently has three components: biochemical (also known as ‘bloodspot’ screening) for serious and treatable metabolic and endocrine conditions; congenital heart disease by measuring newborn oxygen saturation; and hearing screening to detect congenital hearing impairment.
What is the history of newborn screening?
In the 1960s, Robert Guthrie pioneered a technique to test newborn babies for phenylketonuria (PKU). This metabolic condition is a cause of intellectual disability. Treatment with dietary modification is simple and effective and, if started in the newborn period, results in normal development.²
Newborn screening for PKU started in Australia in the late 1960s; congenital hypothyroidism was added in 1977, and cystic fibrosis was added to the panel in the 1980s. Limited changes were made until the early 2000s when a new technology, tandem mass spectrometry, was introduced. This measures levels of proteins in the blood and enables screening for over 20 severe and treatable metabolic conditions.³ Currently, in Australia, approximately 27 conditions are included in newborn bloodspot screening, with some variations by state.⁴
The principles that underpin newborn screening, and health screening generally, were outlined by Wilson and Jungner in 1968.⁵ The key elements for a condition to be included are: having a significant impact on health; available treatment or intervention; and accurate and reliable test to detect the condition. While these principles continue to be recognised, advancing technology requires other elements to be considered.
Genomics in newborn screening
For many years, there has been debate about the use of genomics in a public health setting for newborn screening. On one hand, understanding a baby’s DNA from the time of birth will allow access to accurate and preventative healthcare. On the other hand, expense, data management and consent have consistently been raised as concerns. We are now at the tipping point of this becoming a reality as the cost of genomic sequencing has decreased and our understanding of how to interpret the data generated has improved.⁶
Genomics is the study of our DNA, the code that provides the instructions for our body to develop, grow and function. Spelling mistakes (variants) in DNA can cause rare disease.⁷ Using genomic sequencing would allow hundreds of additional conditions to be screened in newborns. It is not anticipated that genomic sequencing would replace current newborn screening, rather it would be used to enhance and expand the program. This is because both tests have strengths and weaknesses at detecting different conditions.⁸
Impact of genomics on care
In clinical practice, genomics is increasingly used to diagnose and direct management. There is good evidence for benefit in the setting of critically ill newborns suspected of having a genetic condition,⁹ infantile epilepsy,10 children with intellectual disabilities11 and for congenital deafness.12
The use of genomics in the neonatal intensive care setting provides powerful examples of how individuals, families and health care systems might benefit from genomic newborn screening.
Oliver was born after a routine pregnancy in good condition. He established breastfeeding and was discharged home. At 6 weeks of age he attended the emergency department with abnormal movements and colour change. Oliver was admitted to the neonatal intensive care unit with seizures. He was commenced on an anti-epileptic medication. There was no improvement in his condition and he was treated with an additional medication. His parents were overwhelmed and very worried about him. Rapid genomic testing was organised 6 days into Oliver’s admission and revealed two pathogenic variants in the gene PNPO. This confirmed that Oliver’s seizures were due to a deficiency in the B vitamin pathway and he was commenced on the safe and inexpensive medications thiamine and biotin. Oliver became seizure free and his other medications were stopped. He was discharged home 3 weeks after his admission and continues to grow and develop normally. His parents have used IVF with pre-implantation genetic diagnosis to have two more children without this condition.
In this example, Oliver had a significant change in clinical care as the result of his genomic test. If he had genomic sequencing prior to becoming unwell with seizures, he could have commenced treatment prior to, or at the time of, seizure onset and potentially avoided a hospital admission. Preventing a hospital admission has many benefits at an individual, family and societal level. Delivering on the promise of precision therapy, treating an individual with accurate knowledge on the cause of their condition is the biggest opportunity provided by integrating genomic sequencing into screening.
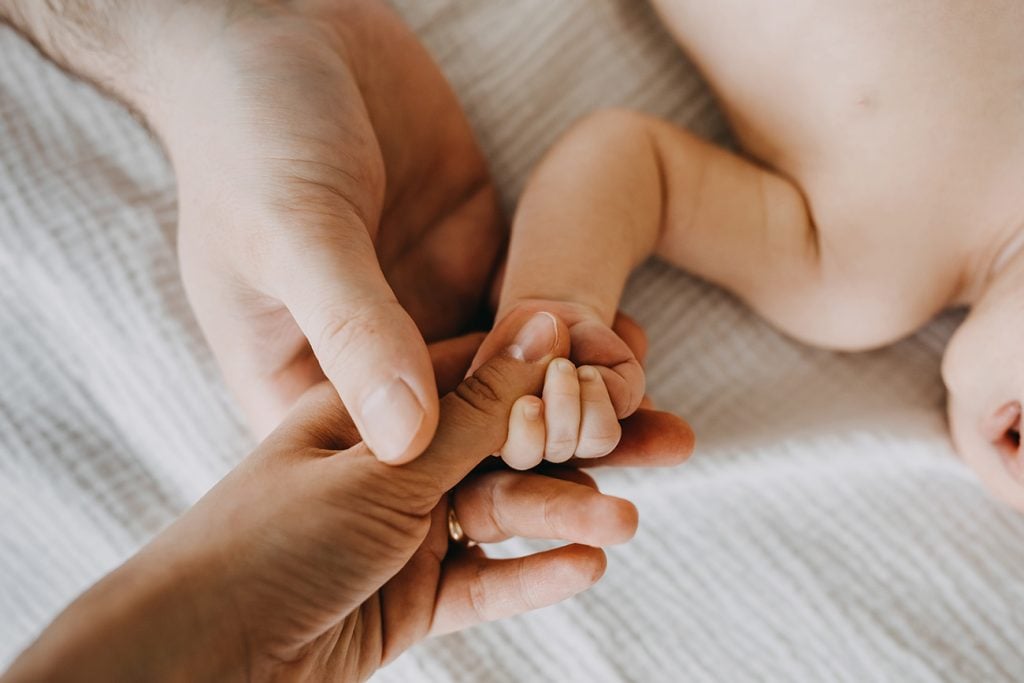
Should genomics be used in screening?
Obstetricians might have encountered genomic sequencing in the setting of reproductive genetic carrier screening.13 This identifies couples that carry variants, which are usually harmless to them, but can cause significant health problems in children if inherited from both parents (autosomal recessive), or from mothers for select (X-linked) conditions. Genomic newborn screening would additionally screen for conditions that are not inherited, but are due to new (de novo) variants in a child, with a particular focus on treatable disorders.
While introducing genomics into screening provides the prospect of significantly increasing the conditions screened, access to genetic counsellors and other health professionals with experience in genomics is essential. Screening programs are not effective when just the test is available, they need to have public health program infrastructure so that people are appropriately informed, followed up and supported through the process. This will allow clinicians and families to have accurate and digestible information and access to support when needed.
What’s happening in Australia and internationally?
In the United States, public funding supports ongoing research into how to use genomic sequencing in newborn screening in the safest and most effective way.14 In 2022, Federal funding from the Medical Research Futures Fund (MRFF) was announced to support research into genomics in newborn screening in Australia. Five projects received funding and the researchers have formed a consortium (GenSCAN) to collaborate and maximise the impact of their respective research findings.
BabyScreen+ is the project currently recruiting in Victoria. BabyScreen+ will provide whole genome sequencing to 1000 newborns for just over 600 childhood-onset, treatable diseases. This pilot study will investigate the feasibility of this test from end to end and measure how acceptable it is to expecting and new parents, as well as the general public. (Lunke, in press) Internationally, the International Consortium on Newborn Sequencing (ICoNS) has been established to similarly strengthen and share results from international research pilots, some of which aim to include 100,000 newborns.
Other considerations for genomic newborn screening
The interpretation of genomic data is highly complex and interpretation of variants changes as our knowledge increases over time. In order to minimise uncertainty and false positive results, genomic newborn screening tests generally only report variants that are highly likely to cause a condition in the screened baby. Combined with evolving therapies and technologies, this requires the genomic newborn screening test to be dynamic in responding to changes in knowledge.
The complexity means that there is still substantial human input into interpreting genomic data and therefore high cost. For a public health program to be successful, it needs to be at low cost to demonstrate benefit to the whole community. Other important considerations are: equitable access to testing and appropriate follow up, funding, and how to ensure the health care system is able to cope with this new test and the flow-on impacts.15
While direct-to-consumer genomic tests for the healthy population are becoming available to those who can afford them, this model has the danger of being poorly regulated and not evidence based, creating a risk for unnecessary investigations and parental anxiety.16
One of the aims of the research pilots, including BabyScreen+, is to help clarify if genomic sequencing is suitable for use as part of public health programs, or whether it is more appropriate as an independent test that parents can access if they want to have additional health information.
What do I need to know for my patients?
Currently, routine newborn screening remains unchanged and provides an exemplar for good public health programs. Research consistently demonstrates that expectant parents and the general public want to do the best for children and provide this as a reason they would support expanding newborn screening.17 Pilot studies will help pinpoint how this new technology can be used to provide benefit for families and minimise harm or unnecessary worry. This research will also answer important questions about how to implement genomic screening by understanding the perspectives of healthcare providers involved in the proposed programs. Collectively, information gained from this research will establish a solid foundation for future development of newborn screening, which will ultimately lead to early diagnosis for babies with serious but treatable genetic conditions.
References
- Therrell BL, Padilla CD, Loeber JG, et al. Current status of newborn screening worldwide: 2015. Semin Perinatol 2015;39(3):171–187. doi:10.1053/j.semperi.2015.03.002
- Levy HL. Robert Guthrie and the trials and tribulations of newborn screening. Int J Neonatal Screen 2021;7(1):5. doi:10.3390/ijns7010005
- Schulze A, Lindner M, Kohlmüller D, Olgemöller K, Mayatepek E, Hoffmann GF. Expanded newborn screening for inborn errors of metabolism by electrospray ionization-tandem mass spectrometry: results, outcome, and implications. Pediatrics 2003;111(6 Pt 1):1399–1406. doi:10.1542/peds.111.6.1399
- Australian Government, Department of Health. Newborn bloodspot screening. [accessed July 2021]. Available at https://www.health.gov.au/health-topics/pregnancy-birth-and-baby/newborn-bloodspot-screening
- Wilson JMG, Jungner G. Principles and practice of screening for disease. Geneva: World Health Organization; 1968.
- Stark Z, Scott RH. Genomic newborn screening for rare diseases. Nature Rev Genet 2023;24(11):755–766. doi:10.1038/s41576-023-00621-w
- Donoghue S, Downie L, Stutterd C. Advances in genomic testing. Aust Fam Physician 2017;46(4):200–204.
- Wojcik MH, Zhang T, Ceyhan-Birsoy O, et al. Discordant results between conventional newborn screening and genomic sequencing in the BabySeq Project. Genet Med 2021;23(7):1372–1375. doi:10.1038/s41436-021-01146-5
- Lunke S, Bouffler SE, Patel CV, et al. Integrated multi-omics for rapid rare disease diagnosis on a national scale. Nat Med 2023;29(7):1681–1691. doi:10.1038/s41591-023-02401-9
- D’Gama AM, Mulhern S, Sheidley BR, et al. Evaluation of the feasibility, diagnostic yield, and clinical utility of rapid genome sequencing in infantile epilepsy (Gene-STEPS): an international, multicentre, pilot cohort study. Lancet Neurol 2023;22(9):812–825. doi:10.1016/s1474-4422(23)00246-6
- Stark Z, Tan TY, Chong B, et al. A prospective evaluation of whole-exome sequencing as a first-tier molecular test in infants with suspected monogenic disorders. Genet Med 2016;18(11):1090–1096. doi:10.1038/gim.2016.1
- Downie L, Halliday J, Burt R, et al. Exome sequencing in infants with congenital hearing impairment: a population-based cohort study. Eur J Hum Genet 2020;28(5):587–596. doi:10.1038/s41431-019-0553-8
- Archibald AD, McClaren BJ, Caruana J, et al. The Australian Reproductive Genetic Carrier Screening Project (Mackenzie’s Mission): design and implementation. J Pers Med 2022;12(11):1781. doi:10.3390/jpm12111781
- Berg JS, Agrawal PB, Bailey DB, Jr, et al. Newborn sequencing in genomic medicine and public health. Pediatrics 2017;139(2):e20162252. doi:10.1542/peds.2016-2252
- Friedman JM, Cornel MC, Goldenberg AJ, Lister KJ, Senecal K, Vears DF. Genomic newborn screening: public health policy considerations and recommendations. BMC Med Genomics 2017;10(1):9. doi:10.1186/s12920-017-0247-4
- Wolf SM, Ossorio PN, Berry SA, et al. Integrating rules for genomic research, clinical care, public health screening and DTC testing: creating translational law for translational genomics. J Law Med Ethics 2020;48(1):69–86. doi:10.1177/1073110520916996
- Downie L, Halliday J, Lewis S, Amor DJ. Principles of genomic newborn screening programs: a systematic review. JAMA Netw Open 2021;4(7):e2114336. doi:10.1001/jamanetworkopen.2021.14336
Leave a Reply